Agricultural machines powered by electric motors powered by so-called “lithium batteries” are on the rise, components that can however be characterised by extremely different chemical compositions and therefore equally different performances. In these pages, a review of the most widespread types on the market, developed thanks to the collaboration with the online magazine “NewsAuto.it”
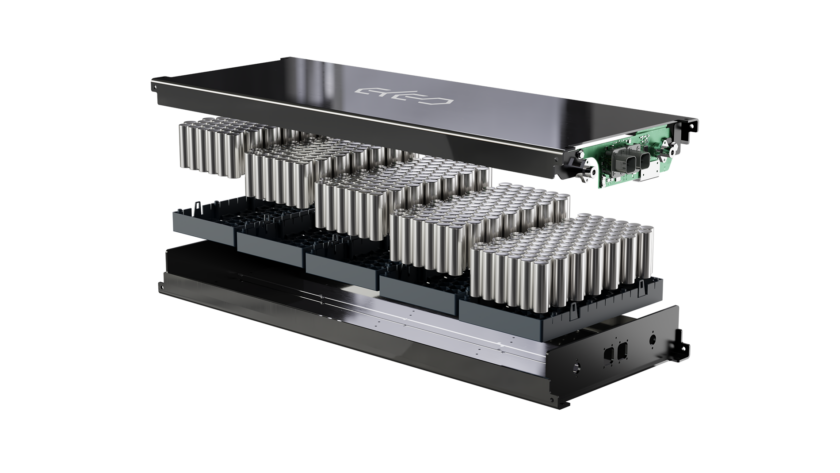
The rise of electric-powered agricultural machinery has led to increased reliance on so-called “lithium batteries.” However, these batteries can feature vastly different chemical compositions, leading to significant performance variations. In this article, created in collaboration with the online magazine “NewsAuto.it,” we explore the most common lithium battery types available on the market.
Lithium Manganese Oxide (LiMn2O4) – Lmo
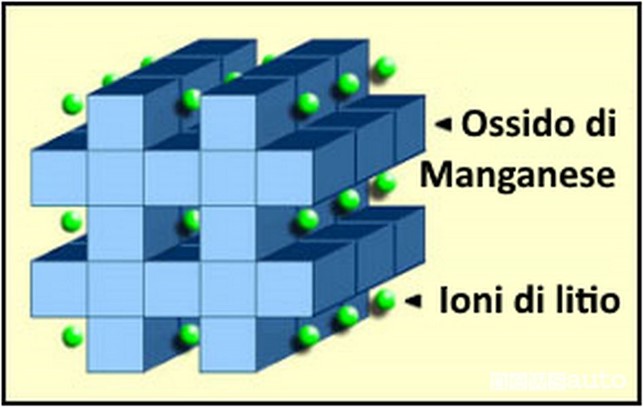
This battery type uses lithium manganese oxide at the cathode, structured in microcrystals that form a three-dimensional network to facilitate electron flow and minimize electrical resistance. This architecture allows for better thermal stability and enables rapid charging along with high discharge currents. However, its energy storage capacity is about one-third lower than lithium-cobalt batteries, and its lifespan is shorter. To enhance capacity, lithium-manganese cells are often combined with lithium-nickel-manganese-cobalt (NMC) cells.
Lithium Cobalt Oxide (LiCoO2) – LCO
Commonly used in smartphones, laptops, and tablets, LCO batteries feature a cobalt oxide cathode with a layered microstructure that facilitates lithium-ion movement and a graphite anode. These batteries offer high energy density but have a shorter lifespan and lower thermal stability. Additionally, they suffer from the “SEI” (Solid Electrolyte Interface) phenomenon, where a solid lithium deposit forms on the anode, increasing resistance to charging—especially during fast charging and at low temperatures. Modern versions incorporate nickel, manganese, and aluminum to improve longevity and reduce costs. Due to the high cost of cobalt and advancements in other lithium chemistries, LCO batteries are gradually being replaced by lithium-manganese alternatives.
Lithium Nickel Manganese Cobalt Oxide (LiNiMnCoO2) – Nmc
The most common chemistry in electric vehicles, NMC batteries optimize performance by balancing energy density and power output. The combination of nickel and manganese allows for high specific energy while maintaining a stable crystalline structure. They are available in different compositions, such as “1-1-1” (equal parts nickel, manganese, and cobalt) and “5-3-2” (higher nickel content). Manufacturers are increasingly reducing cobalt in favor of nickel to lower costs, despite a reduction in cell voltage. These versatile batteries are used in both electric traction and energy storage applications.
Lithium Iron Phosphate (LiFePO4) – Lfp
Using iron phosphate as the cathode, LFP batteries provide low resistance, high discharge currents, long cycle life, and excellent thermal stability. Nanotechnology enhances their safety and resistance to high voltages. However, they have lower energy density compared to cobalt-based batteries and a higher self-discharge rate, which can cause imbalance over time. This issue is managed with advanced electronic controls, increasing complexity and cost. Additionally, LFP cells are highly sensitive to moisture, requiring adequate protection. Despite these drawbacks, they are commonly used as lead-acid battery replacements in automotive applications.
Lithium Nickel Cobalt Aluminum Oxide (LiNiCoAlO2) – Nca
Similar to NMC batteries, NCA chemistry provides high specific energy, good power output, and long lifespan. However, they have higher safety risks and production costs. NCA batteries represent the latest evolution of lithium-cobalt technology, with aluminum added for greater chemical stability. Due to their high potential, they are considered key candidates for future electric propulsion systems.
Lithium Titanate (Li2TiO3) – LTO
LTO batteries use lithium titanate at the anode, featuring a microcrystalline structure. They can be paired with lithium-manganese or lithium-manganese-cobalt cathodes. These cells allow for ultra-fast charging and can withstand discharge currents up to ten times their nominal capacity. They offer excellent low-temperature performance, long lifespan, and high thermal stability. Unlike conventional lithium batteries, LTO cells do not suffer from lithium plating during rapid charge/discharge cycles in extreme temperatures. However, they have lower energy density and higher production costs.
Solid-State Batteries: The Future of Electric Propulsion
Solid-state batteries represent a significant leap forward in electric vehicle technology. Unlike traditional lithium-ion batteries, which consist of a liquid electrolyte, solid-state batteries use a solid electrolyte, improving energy storage capacity and reducing charging times. Traditional lithium-ion cells contain four elements: anode, cathode, liquid electrolyte, and separator. Solid-state batteries simplify this design to just three elements: anode, cathode, and solid electrolyte, which acts as both an ionic conductor and separator.
Solid-state electrolytes can be polymeric, ceramic, or hybrid. Polymeric electrolytes enable ion transport through their molecular structure and can be synthesized in flexible thin films. Ceramic electrolytes use crystalline or glass structures that facilitate ion movement, even at room temperature, while maintaining excellent chemical stability. Hybrid electrolytes combine properties of both polymeric and ceramic materials for improved ionic conductivity and flexibility.
Since solid electrolytes occupy less space than liquid ones, these batteries achieve higher energy density while maintaining a compact size. They also allow each cell to deliver up to five times more current than conventional lithium-ion batteries while doubling energy density. Additionally, solid-state batteries are significantly safer, as they mitigate risks of fire or explosion and offer greater longevity due to their stable electrolytes. This enables them to endure more charge/discharge cycles without substantial performance degradation, making them ideal for long-term, high-performance applications.
Despite these advantages, production costs remain high—currently almost twice that of traditional lithium-ion batteries with liquid electrolytes. However, as technology advances, solid-state batteries promise to bridge the gap between electric and internal combustion vehicles by enhancing driving range and reducing charging times.
How a battery works
Batteries work on the basis of an electrochemical reaction that occurs between two electrodes, the cathode, positive, and the anode, negative, immersed in an electrolyte that connects the electrodes together, allowing the passage of ions from one to the other. During discharge, a flow of electrons is created that travels from the anode to the cathode through the external circuit, producing usable electrical current. At the same time, and inside the battery, a flow of ions is created that move in the electrolyte from the anode to the cathode to maintain the charge balance. During charging, the process is reversed: an external current source forces the electrons to flow towards the anode, accumulating chemical energy that can be used later. This process is made possible only by specific materials that allow the flow of electrons and ions in a controlled and reversible manner, as in the case of rechargeable batteries.
Title: Lithium batteries: operation and characteristics
Translation with ChatGPT